Superconductors are materials that can conduct direct current with zero resistance, when they are cooled below a specific temperature known as the critical temperature or Tc. In other words, when electric current is passed through a superconductor, it will flow without experiencing any resistance to flow of current, unlike normal conductors which will encounter resistance and convert some of the electrical energy into heat. Resistance to flow of current is found in metal conductivity also in semiconductors while in superconductor there will not any resistance.
Superconductivity was originally discovered in 1911 by Dutch physicist Heike Kamerlingh Onnes while he was researching the conductivity of various materials at low temperatures or Tc. He discovered that the resistance of mercury dropped to zero when it was cooled to a temperature of 4.2 Kelvin (-452.07°F). This was first time that the phenomenon of superconductivity had been observed, and it sparked a great deal of interest and research in the field.
He observed that the resistance of some materials, such as mercury and lead, would suddenly become zero when cooled to a specific temperature known as the critical temperature. The critical temperature differs depending on the material, but it is usually quite low, ranging from -243 to -138 degrees Celsius (-405 to -216 degrees Fahrenheit).
Mercury has been one of the most extensively investigated superconducting materials since its initial discovery of superconductivity. Mercury is a desirable material for a range of applications since it is very simple to deal with and has a comparatively high critical temperature in comparison to other superconducting materials.
Here are some properties of superconductors:
- Zero resistance to the flow of electricity
- The ability to exclude magnetic fields (the Meissner effect)
- The formation of pairs of electrons (Cooper pairs)
- The ability to conduct electricity perfectly
- The need for extremely low temperatures in order to exhibit superconductivity
- The potential for a wide range of applications, including in MRI machines and particle accelerators, magnetic levitation etc.
How superconductivity Works?
The formation of pairs of electrons known as Cooper pairs, which may pass through a material without encountering resistance, is the fundamental mechanism behind superconductivity. In a typical conductor, the contact between the electrons and the material’s atoms prevents the transport of electrons. The electrons can form these pairs and pass through a superconductor without encountering this resistance.
One of the most striking properties of superconductors is their ability to exclude magnetic fields, a phenomenon known as the Meissner effect. This occurs because the electrons in a superconductor can move without resistance, creating a current that cancels out the external magnetic field. As a result, a superconductor can be levitated above a magnet, as the magnet’s field is unable to penetrate the superconductor.
Superconducting metals and their critical temperatures:
- Aluminium: Aluminium becomes a superconductor when cooled to about 1.2 K (-272°C).
- Lead: Lead becomes a superconductor when cooled to about 7.2 K (-266°C).
- Tin: Tin becomes a superconductor when cooled to about 3.7 K (-269°C).
- Mercury: Mercury becomes semiconductor when cooled down to about 4.2 Kelvin or (-452.07°F).
- Niobium-Titanium: Niobium-Titanium alloys become superconductors when cooled to about 9.3 K (-264°C).
- Niobium-Tin: Niobium-Tin alloys become superconductors when cooled to about 15 K (-258°C).
- Nickel: Nickel becomes a superconductor when cooled to about 0.9 K (-272°C).
- Iron: Iron becomes a superconductor when cooled to about 0.5 K (-273°C).
- Zinc: Zinc becomes a superconductor when cooled to about 0.85 K (-272°C).
- Magnesium diboride (MgB2): Magnesium diboride becomes a superconductor when cooled to about 39 K (-234°C).
- Uranium: Uranium becomes a superconductor when cooled to about 0.016 K (-273°C).
- Plutonium: Plutonium becomes a superconductor when cooled to about 0.02 K (-273°C).
- Cerium: Cerium becomes a superconductor when cooled to about 0.45 K (-272°C).
- Lanthanum: Lanthanum becomes a superconductor when cooled to about 0.45 K (-272°C).
- Yttrium: Yttrium becomes a superconductor when cooled to about 0.5 K (-273°C).
- Samarium: Samarium becomes a superconductor when cooled to about 0.5 K (-273°C).
- Erbium: Erbium becomes a superconductor when cooled to about 0.5 K (-273°C).
- Dysprosium: Dysprosium becomes a superconductor when cooled to about 0.5 K (-273°C).
- Holmium: Holmium becomes a superconductor when cooled to about 0.5 K (-273°C).
- Thulium: Thulium becomes a superconductor when cooled to about 0.5 K (-273°C).
Note: The values may vary, these values are collected from internet.
Copper and superconductivity
At any temperature, copper is a metal that lacks superconductivity. When chilled below a specific temperature, referred to as the critical temperature, a material exhibits zero electrical resistance and the capacity to emit magnetic fields, a phenomenon known as superconductivity. Copper does not exhibit superconductivity because it does not have a critical temperature at which it turns into a superconductor.
Cuprates superconductors
High-temperature superconductors, which are materials that can become superconducting at temperatures higher than previously known superconductors, have been the focus of recent research. These materials are usually ceramic compounds made of copper and oxygen, called cuprates.
In 1986, a team of researchers at IBM found a cuprate that became superconducting at a temperature of -135°C (-211°F), which was much higher than the critical temperature of any previously known superconductor. Since then, other high-temperature superconductors with critical temperatures ranging from -110°C to -70°C (-166°F to -94°F) have been discovered.
While these materials have the potential to make superconductivity more practical and widespread, they are not yet widely used due to the difficulties in working with them and a lack of complete understanding of how they superconduct.
Applications of superconductivity/
superconductors:
Superconductors have the potential to be used in a variety of applications, such as electrical power transmission, medical imaging, and maglev trains. However, their usefulness is limited because of their critical temperature, as most superconductors must be cooled to very low temperatures in order to function. This can be costly and impractical, especially for large-scale applications.
One of the most promising applications of superconductors can be used for is electrical power transmission. Normal power transmission lines experience resistance, which causes energy loss as heat. This means that a large amount of energy must be generated greater than the requirement in order to transmit a given amount of power over long distances without reduction in power. Superconducting transmission lines, on the other hand, would be able to transmit power with zero loss, making them much more efficient. However, this technology is far fetched for us and not easy to implant.
Another potential use for superconductors is in medical imaging through MRI machines. These machines use strong magnetic fields to generate detailed images of the inside of the human body, but these fields can be harmful to certain medical implants like pacemakers. Using superconducting materials could potentially create MRI machines with weaker, safer magnetic fields for use with implants.
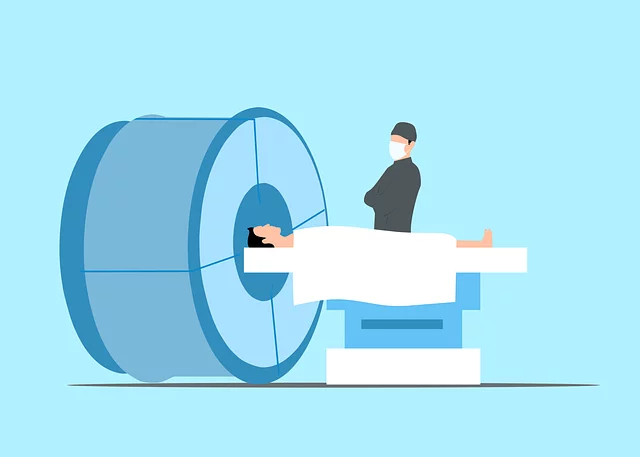
Additionally, superconductors are being examined for use in maglev trains, which use powerful magnets to lift and move the train along a track. Using superconducting materials in the magnets could potentially make the trains more efficient and faster by allowing the magnets to produce a stronger field with less energy.
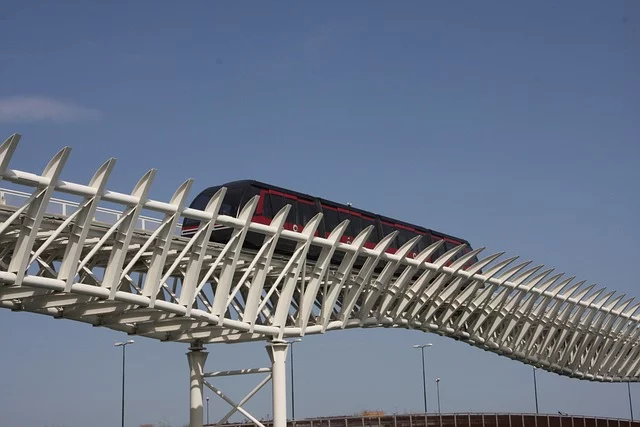
Challenges associated with superconductors / superconductivity
The key challenge of the superconductivity is the fact that they only show their zero-resistance behaviour at very low temperatures. This means that in order to use superconductors in any kind of practical applications, they must be cooled to extremely low temperatures. This can be done using liquid helium, which has a boiling point of 4.2 K (-452.07°F), the same temperature at which mercury becomes a superconductor.
Researchers developing new materials that can exhibit superconductivity at higher temperatures to make superconductivity practical and economical. Maybe someday scientists would be able to overcome problems associated with semiconductors that will make superconductivity practical and will be used for making newer technologies.